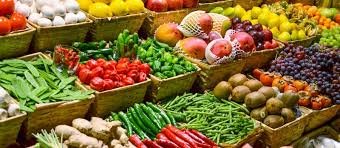
Prof. K C Bansal, Board Member of the Global Plant Council and Senior Consultant, Alliance of Bioversity International and International Center for Tropical Agriculture (CIAT) shares his views on the ‘Effective use of Genome editing in agriculture’. Edited excerpts – Part -I
The Nobel Prize for Chemistry, 2020 has been awarded to Emmanuelle Charpentier and Jennifer Doudna for developing the Genome Editing technology- CRISPR-Cas9. In some countries like the US, biotech companies have started to apply CRISPR[1]Cas9 in agriculture. In an interaction with AgroSpectrum, Prof. K C Bansal, Board Member of the Global Plant Council and Senior Consultant, Alliance of Bioversity International and International Center for Tropical Agriculture (CIAT), Former Director,ICAR-National Bureau of Plant Genetic Resources, New Delhi shares his views on the ‘Effective use of Genome editing in agriculture’. Edited excerpts –
- The 2020 Nobel Prize has been awarded to the developers of genome editing. How do you see the potential of this technology for agriculture?
It’s indeed quite heartening to learn that the 2020 Chemistry Nobel Prize has gone to the Genome Editing technology developed by Emmanuelle Charpentier and Jennifer Doudna. No doubt, this technology has tremendous potential as already evident from the literature on basic biological research, human therapeutics and agriculture.
As a scientist engaged in genetic modification of crops for over two decades, I see a great opportunity ahead for another revolution in agriculture, particularly by developing smart crops with desired attributes to bring sustainability to crop production. Genome editing has already created crops with improved traits that were hitherto, technically speaking, difficult through conventional breeding or genetic engineering. For instance, the development of genome-edited rice with improved growth and high yield combined with high tolerance to drought by mutating ABA receptors, and drought-tolerant maize developed by mutating the negative regulator of ethylene response. Similarly, tomato has been made resistant to the powdery mildew fungal pathogen through loss-of-function mutations in the wild-type alleles of the Mlo gene. Another example is the generation of genome-edited rice with broad-spectrum resistance to bacterial blight by mutating the sucrose transporter gene promoters.
Notably, all this has been achieved by modifying only a few bases/nucleotides in a precise and targeted manner, as against random mutagenesis attempted in the past through irradiation or chemical mutagens, which resulted in the development of thousands of crop varieties that were released in several countries including India. The genome-edited crops developed with minor targeted genetic modifications in their native genes remain indistinguishable from their non-edited counterparts. There are several complex agronomic traits that need immediate attention, and now we can even use CRISPR-based multiplex genome editing for improving the complex traits with relative ease.
More importantly, genome editing will allow enhanced use of crop diversity by bringing novel resistance genes/alleles from crop wild relatives and landraces to the cultivated gene pool for developing high-yielding climate-smart crops with enhanced resistance to emerging pests and pathogens, and tolerance to multiple stresses, such as drought, high temperature and salinity. Thus, I would say that genome or gene editing mediated by the CRISPR-Cas system has emerged as a game-changer with a great promise to develop high-yielding and climate-resilient crops.
Also, this technology is here to stay and India must take as much benefit as possible for the welfare of smallholder farmers, consumers and society at large, and for a clean and green environment.
- What is genome editing and how does it differ from the classical way of genetic engineering?
Genome editing technology is based on an existing bacterial immune system, and allows modifications in an organism’s genome, be it insertions/deletions or substitution of certain bases or even inserting a full-length foreign gene at the predetermined genomic site in a precise and targeted manner. Conventional genetic engineering on the other hand, either Agrobacterium-mediated or biolistic-driven, brings in random integration of the foreign gene in the genome. This necessitates GM (genetic modification) crops to go through a lengthy development process as well as clearance through a complex regulatory regime. Interestingly, the genome-edited crops are accepted in many countries as non-GMOs owing to the absence of foreign DNA in the final product.
Genome editing involves cleavage of the plant’s double-stranded DNA by the CRISPR-associated (Cas) protein. This is followed by repairing of the double-stranded break by the cell’s own machinery through non-homologous end-joining (NHEJ), subsequently introducing variable-length deletions/additions of few nucleotides in the given gene and making it non-functional (knocking out the gene). This way, the genome editing technology allows modification of plants’ own genes and is not dependent on foreign gene insertion, unlike genetic engineering, for incorporating a particular trait. The technology allows targeted substitution of certain nucleotides within the plant’s own gene’s coding sequence or the regulatory elements of a gene, with the use of homologous sequences through a homology-directed repair (HDR) system. And as mentioned before, the technology can be used to precisely insert a full-length foreign gene onto a predetermined genomic location or replace a given gene through the HDR mechanism.